NH3 as a Biosignature
The most common type of planets found are those whose radii placing them between Earth and Neptune in terms of size. This population of smaller planets is divided into two regimes, the Super-Earths and the Sub-Neptunes. The former are thought to have lost their primordial hydrogen rich atmospheres while the latter appear to have retained them, and thus are often referred to as gas dwarf planets. Despite their prolific appearance in other planetary systems, neither population has an analogue within the Solar System, which naturally brings forth questions about their formation and the true nature of these worlds, Furthermore, the unknown conditions on these worlds also present intriguing questions about habitability and the potential to detect biosignatures,
Gas dwarf planets are more amenable targets than Earth-like planets for transit observations because of their larger radii and lighter atmospheres. Due to the hydrogen dominated atmosphere, gas dwarf planets have different atmospheric chemistry to those dominated by molecules such as N2 or CO2. The dominance of the hydrogen creates a reducing chemistry, causing other elements to preferentially react with hydrogen to produce molecules such as water (H2O), ammonia (NH3), and methane (CH4). This is in contrast with the oxidizing chemistry that exists on the inhabited Earth. We, therefore, expect different biosignatures in gas dwarf planets.
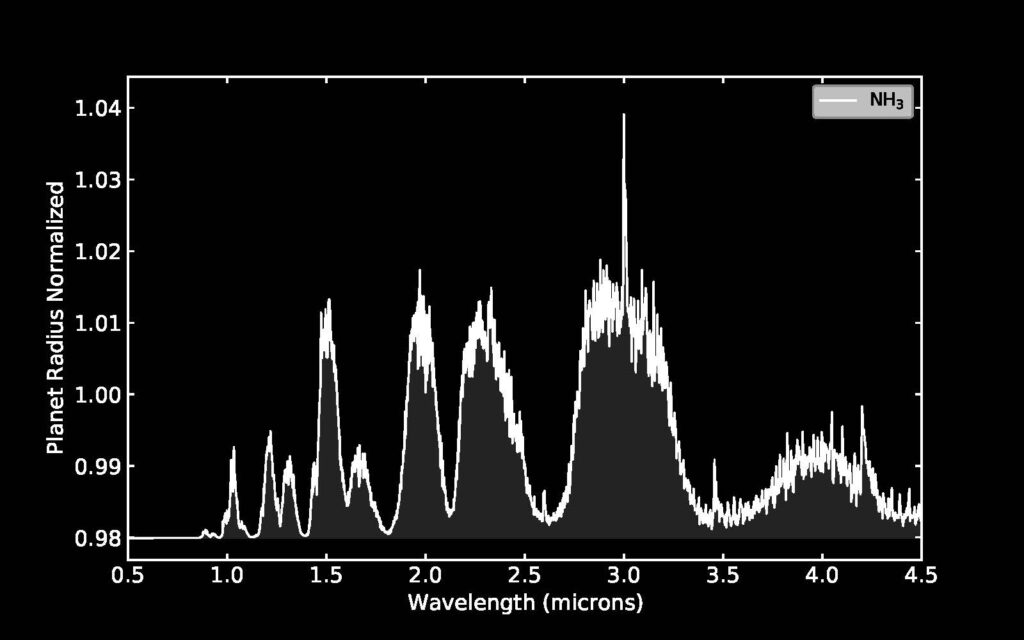
Sara Seager first proposed NH3 as a biosignature gas in a H2 and N2 dominated atmosphere, nicknamed a “cold Haber World”. NH3 is a strong candidate as a biosignature for the several reasons. First, the reaction that produces NH3 from N2 and H2 is exothermic, i.e., releasing energy that can be harnessed by life to support metabolism. Second, this reaction requires high temperatures (>450 K) and high pressures (>10 bar) in abiotic environments, and therefore the existence of NH3 at low temperatures and pressures in the upper atmosphere implies the existence of a reaction catalyst, potentially developed by life for metabolic processes. Third, NH3 is easily destructible in photochemistry and volcanic environments. Therefore, any NH3 has to be replenished by certain productive processes that potentially involve life.
Twinkle will perform simultaneous spectroscopic coverage of the 0.5 – 4.5 micron wavelength region, which covers many ammonia features as shown in Figure 1. Therefore, the pursuit of searching for potential biosignatures in these worlds is an exciting endeavour for the upcoming Twinkle space mission.
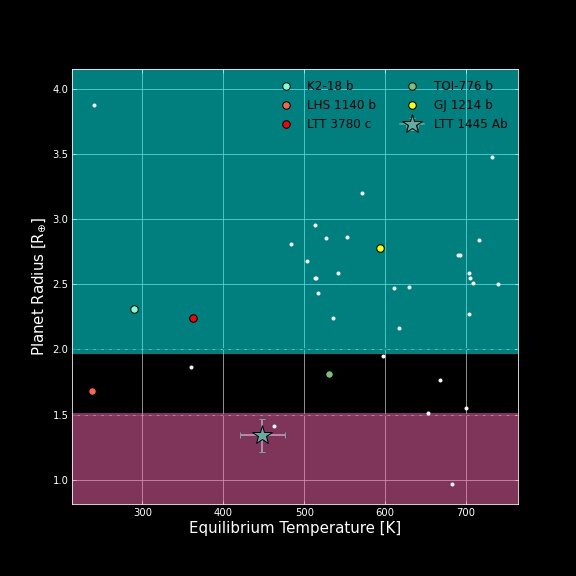
Suitable Planets Within Twinkle Field of Regard
Currently, there are ~ 750 confirmed transiting exoplanets within Twinkle’s field of regard, with many more likely to be found by the Transiting Exoplanet Survey Satellite (TESS). We are currently in the process of finding suitable targets for observations with Twinkle. Ideally, we are searching for planets with a radius between 1.3 – 3.4 Earth radii, an equilibrium temperature < 680 K, whose host star is within around 50 pc of the Earth (Figure 2). The upper radius limit is such that we reduce the chance of the targets having a high enough pressure to produce ammonia abiotically such as in Jupiter. Recent work by Huang et al 2021 explored ammonia as a potential biosignature on terrestrial like-planets and so we are exploring a lower boundary of terrestrial-like planets with potentially hydrogen dominated atmospheres. In a study by Nixon and Madhusudhan (2021), they also found that the phase structure of water-rich sub-Neptunes show indications that planets with a primordial envelopes could host H2O in the liquid phase at up to 647 K and pressures of 218 -7 x 10^4 bar. Lastly, the distance of less than 50 pc, is to ensure adequate flux from the host star and planet for transmission spectroscopy with Twinkle. These factors combined will allow us to find ideal targets to search for potential biosignatures in gas dwarfs and potentially terrestrial-like planets.
One of the current targets of interest is the nearby (6.9 pc) planet, LTT 1445 Ab. LTT 1445 Ab is the closest transiting system to orbit an M-dwarf, and is a part of a triple M-Dwarf system. LTT 1445 A b has a radius of 1.38 Re, a mass of 2.2.Me and an effective temperature of round 430 K. Preliminary simulations of observations of LTT 1445 Ab show a favorable signal-to-noise ratio for searching for biosignatures with the Twinkle mission (Figure 3).
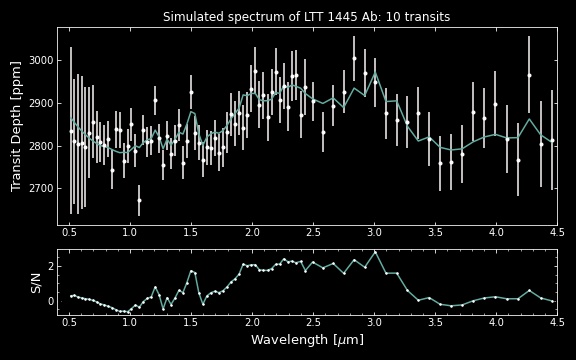
The Full Picture: Synergy with JWST
Twinkle will have a broad visible to infrared wavelength coverage to build on exoplanet science already achieved with ground-based telescopes as well as space-based telescopes such as HST and Spitzer. As a dedicated space-based exoplanet spectroscopy mission, Twinkle will enable the detailed characterization of many exoplanet atmospheres in far greater depth. Additionally, Twinkle can be a highly complementary to the James Webb Space Telescope (JWST) as, while JWST will deliver unprecedented precision, oversubscription is likely to be an issue. Therefore, it is likely to only be used to observe the most exciting targets. To this end, Twinkle can provide low-resolution spectroscopy to provide an initial atmospheric characterization to promote further study or be used to refine planetary and orbital parameters. Combining Twinkle data with the upcoming JWST mission will allow us to achieve a fuller picture of exoplanet atmospheres and bring forth a new dawn of biosignature searches across a wide wavelength of molecular and atomic features to explore.
About the Author
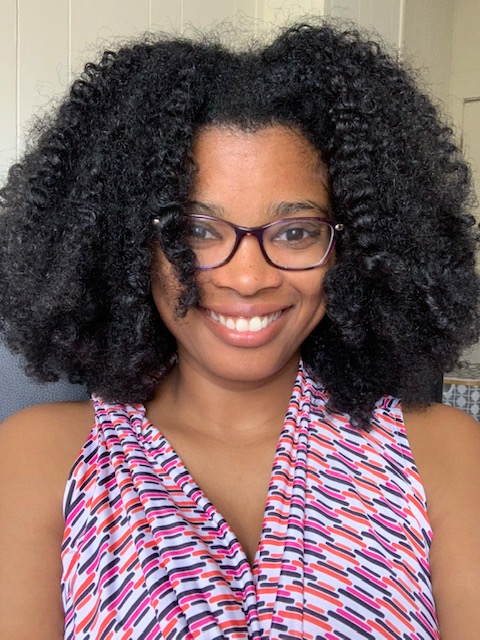
Caprice Philips
PhD Student at Ohio State University
Caprice is a third-year astronomy PhD student at The Ohio State University working with Professor Ji Wang on detectability of biosignatures on gas dwarfs with JWST and Twinkle. She is also a fellow in the LSSTC Data Science Fellowship Program. She received her M.A. in Astronomy from the University of Texas at Austin in 2019 and her B.S. in Physics with a minor in mathematics from The University of Arkansas at Little Rock (UALR) in 2015.